or
Where is everybody?
On the curious fact that we appear to be all alone in this galaxy
Preface
Given how many earth-like planets there probably exist in our galaxy, and how fast life evolved on young earth despite the brutal conditions, one can only assume that there must be life on thousands, millions even billions of other planets. And if there is primitive life on a very large number of planets, chances are good, that there is intelligent or even highly sophisticated, technologically capable life somewhere.
And yet, neither have we proof of technology being somewhere out there, nor of some aliens having visited us. “Then where is everybody”, asked Enrico Fermi already in 1950.
That we do not find proof of alien technology, is, I guess, not so strange: We search mostly for radio waves or other electromagnetic waves. Since they disperse rapidly over distance they are probably to weak to be detected by us. And to detect other forms of communication, like neutrino beams, we lack the technology. Given the probable sparsity of planets where highly technological life can evolve (see below), we are simply to far away from the action to see it. Things might be different near the center of the Galaxy, but that scenario is not included into my thoughts here.
This article focuses mainly on the question, if we ever might meet aliens. Might they come here to visit us? Or could we go out and visit them? There are indeed several arguments that point to a very low probability for meeting E.T. in the foreseeable future or – well – ever. The arguments are the following:
- Sparsity of planets where highly technological life can evolve
I estimate the average distance between solar systems hosting intelligent life to be 50 – 100 light-years. And between those with societies willing and able to engage into interstellar travel 1000 – 2000 light-years. - Issues and motivation in highly technological societies
Only non-peaceful societies will seek contact to or try to colonize other solar systems. Reasonable max-speed is 25% of light speed, resulting in enormous times for preparations and travel, while expected gain is zero. Almost no non-peaceful society will have the surplus and survive long enough to succeed. - Travel costs
Costs itself are high and must be multiplied by a chance of success next to zero. To have a 95% chance that one ship survives a 50 light-year travel (200 years at 25% c) you have to send at least 35 fully equipped and maned ships. For a 95% chance with a 1000 light-year travel you have to send 500 ships. Personal and financiers ought to be hard to find. - Pre-existing life on probable target planets
Due to the extreme needs for fuel there is very little room for defensive equipment. There is virtually no information about what one will meet. Even the most primitive life-forms will have an advantage. - Dangerous consequences!
Due to a time delay in communication of decades and up to millennia there is no way to establish or maintain diplomatic relations. It is much easier and cheaper to destroy a society than to defend yourself from an attack, leading to that both alien societies and colonies grown hostile will prefer a preemptive strike to a life under constant threat. To tell the galaxy that we are here is mere suicide.
In this article I assume that nothing lasts forever, things break and get worn out. Warp drive, wormholes, flying faster that light, levitation, anti gravitation, quantum transportation, stargates, exotic indestructible materials, force fields and other fancy stuff are great for a good science fiction movie, but do not exist in reality.
Simpler calculations are embedded in the text. You find the more complex ones if you follow the numbered links to the Appendix in brackets. Since most calculations are based on pretty rough assumptions, I round most numbers.
I’d like to emphasize that it was not my intention to proof the impossibility of interstellar flight. I love physics, science-fiction and astronomy. I would love to meet some aliens or see their world. I started my calculations out of pure curiosity and I am chocked myself from what I found. It was not my intention to discourage anyone’s ambition or to destroy dreams. I’m really sorry.
When I calculated upon what would happen if we anyways would discover a highly advanced alien civilisation in our neighbourhood (or create one ourselves by sending someone or something to colonize), let’s say 50 light-years away, I found that the best and only reasonable thing to do would be to destroy it as soon as possible! Again, I am really sorry. Not only are we alone, we are doomed to stay alone forever, it seems, and so are all the other civilisations. And if we and they have some wit, keeping quiet and undetected is the only reasonable thing to do.
If you, dear reader, can’t believe I can be right, read my calculations and arguments and please point out to me, where I am wrong. It would be a great relief to me.
1. Sparsity of planets where highly technological life can evolve
From what we can see here on earth, life evolved easily, rapidly and under very tough conditions. Something similar to virus, bacteria and primitive algae should have a good chance to be present in almost every solar system.
However, to evolve from simple to complex life is not so easy. Here on earth it took some 3 billion years to evolve sexual reproduction and other efficient solutions necessary for multi-cellular organisms, bigger than flatworms. I took then another billion years before humans appeared. We can assume that it should take just as long on some other planet. To evolve an ecosystem that produces increasingly sophisticated species, where all sub-systems work together in such a lucky way that they produce ever improving brains and bodies capable of understanding and manipulating the world, takes time. During this time of evolution, conditions must be relatively stable and important minerals and metals need to be recycled all the time.
Many factors determine or prevent that highly technological life may evolve on some of the 200 billion solar systems of our galaxy. Let us try to estimate how many of those we may find near by.
A) The habitable zone
For life to evolve, the home planet must circle its sun at the right distance to be not to cold and not to hot. Most solar systems should have one or several planets within this window of life.
B) Rocky planets
The planet must be rocky, providing a large amount of inorganic minerals and chemicals, as well as basic organic compounds like amino acids, methane etc, and, of course, water. Again in most solar systems with second or third generation suns the inner planets should be rocky planets providing all this.
C) The sun
Suns come in all sizes and colors. 75% of all suns are small, red dwarfs. They shine for many billion years, which gives life a lot of time to evolve. However, they give little light, force the planets in the habitable zone to always face the sun with the same side (like Mercury and our Moon do), having one boiling hot and one icy cold side, and have many other unsuitable properties and chances for high-tech life to evolve are very low. I rule those solar systems basically out.
Too big stars live for too short a time to give high-tech life a chance.
I guess, that about 5% of all solar systems or 10 billion have suns of the right age and size so life had a chance to advance beyond bacteria and flatworms.
D) The moon
Through plate tectonics on Earth necessary raw materials like calcium and carbon are continuously recycled. Without plate tectonics they would have get trapped on the bottom of the oceans and lost for life millions of years ago. Without tectonics volcanic activity stops, when the planet cools, mountains erode down and evolution stops at the level of bacteria in rocks and sediment. Our moon made plate tectonics possible. Maybe even keeps that circle alive. A planet sized moon is needed. The probability for two early planets to collide with just the right speed and at the right angle to form the earth-moon relationship we have, is very low. They shall not crush into each other to merge into one planet, not only touch and move apart. They need to be of the right size and they need to circle each other at the right distance to form a system where the resulting plate tectonics are not to fierce and not to lame. A wild guess from my part is 1 in a thousand solar system might be so lucky that this not only happened, but happened to one of the few planets within the habitable zone. That leaves us with 10 million suitable planets.
E) The planet itself
It needs an electromagnetic field that protects the planet from solar wind that otherwise literally blows away the atmosphere and surface water, long before high intelligence has evolved. Neither Venus or Mars have such a field, and we have no data from exo-planets. I guess that the probability for the one or two planets within a solar systems window of life to have one is, let’s say, 50%. Leaves us with 5 million planets.
F) Climate stability
For intelligence or even technology to evolve, the planet must have a stable climate for a long time. Earth is hit by a major meteor once every 50-100 million years. The dinosaurs, on the brink of developing higher intelligence, where wiped out 65 million years ago. It took intelligence 60 million years to come back again. If the hit-rate is slightly higher, the development of high intelligence might never happen. Other factors may be an unsuitable tilt of the planets axis, atmospheric instability or unsuitable composition, too rapid cycles of ice ages, droughts and other climate changes. Or evolution might bring forth some less intelligent but very hardy and dominant specie, surviving all climate instabilities but suppressing others species etc. My guess is that the evolution of intelligence is prevented or reset over and over again on maybe half of all probable life-hosting planets. That leaves us with 2,5 million planets or roughly 1 in 80 thousand.
If solar-systems that could host highly intelligent life are distributed evenly throughout our neighborhood in the galaxy the next one may be about 160 light-years away (1). However, it does not mean, that there are high-tech lifeforms during the time we are looking for them, or we are here, when they look for us.
2. Issues and motivation in highly technological societies
Any society faces the question of how to organize itself. On a spectrum of possibilities there are, however, basically two ways: diverse or homogeneous. Regardless the individuals are biological, trans-biological, cyborgian or artificial. Regardless their structure is hierarchic or has some other form. And regardless there are real individuals or all beings form some mishmash.
To explore and conquer other worlds requires the accumulation of large amounts of resources around a few individuals as well as the willingness to use force against probable hostile inhabitants of the new worlds in order to survive.
In a homogeneous society each individual of the population will have an equal share of power and resources, big enough to survive and live well, but not big enough to somehow use it to dominate other individuals. Such a society can be stable for millions of years. All activity will just suffice to survive but will not make much fuzz detectable for us. There will be an inclination to avoid conflict, and therefor no inclination to contact other civilizations. All will or wish to fight with and dominate others will have washed out from each individuals genes (what ever form these may have). There will be no motivation to organize the huge enterprise of interstellar flight, unless the existence of the society is threatened, maybe because their sun is running out of fuel. Since that happens very seldom it would be extremely lucky if we caught a glimpse of the few thousand years it might take that society to leave its home planet, compared to the millions of years it might have existed quietly. The probability that they might come to us is even smaller. Most probably they would move to their new home and continue to live there quietly. So the chances for us to detect or even meet a peaceful and homogeneous alien society are next to zero.
Leaves us with the diverse societies. They are, to put it short, unstable and short lived. Driven by competition and egoism, most individuals or sub-groups will only work together to achieve their own goals. What ever way one turns it, if some aliens have a society that allows a somehow selected group to accumulate and rule over large amounts of power and resources, there will be turbulence, intrigues, conspiracies and internal wars that lead to frequent revolutions, break downs, even start-all-overs. Remixing the genes or code, never allowing an elite to truly separate from ‘the people’.
Throughout the universe the driving force to bring forth new species, biological as well as artificial, must be evolution. Although Darwin described evolution as “survival of the fittest” it really is “extinction of the most unfit”. That means that survivors are just fit enough not to be extincted, but far, far from perfect. Thus evolution stops or drifts away into confusion as soon as there is no competition to evolve against. That in turn means that societies are far from perfect and therefor prone to collapse every now and than due to plain stupidity of one sort or other.
Of course, given the 2,5 million solar-systems where highly intelligent societies might evolve, there might occur times and circumstances where even a divers society goes through a long enough period of stability and unity, where each individual is happy to exist on his/her/its place in the hierarchy for millennium after millennium, pushing wealth upwards, not demanding a change until some top elite or selected group has gathered the knowledge and resources needed to develop the high-tech needed to take to the stars.
I estimate that seen over the life-span of one million years, there is only a window of one thousand years where some hypothetical aliens have the stability necessary to bring together the will and resources to explore deep space, before they either collapse again, loose interest or turn into a peaceful quiet society without the wish to travel.
That means that from that 2,5 million possible societies, we are left with only maybe 2,5 thousand or one in 80 million. With even distribution our next smart neighbor building a rocket right now lives 1600 light-years up or down the spiral arm.
If our alien society develops the technology, will and economy to embark on such an enterprise it would face two obstacles: Travel costs and life already thriving on the new planet.
3. Travel costs
Colonizing new solar-systems in the galaxy is very different from colonizing a new land on the same planet. Let’s imagine a population of rats, living on some meadow. They reproduce and multiply and after some time the meadow becomes overcrowded. Every single rat can run, let’s say, half a kilometer from the burrow to feed and run back home. With the population constantly growing and resources constantly dwindling, each rat will feel, that running back home becomes less and less fun. Fights within the group, growing hunger, flies, piles of excrement and so on. Some rats will start to consider to run further away instead of running home. It’s within their capacity. Taking some friends along, chances for survival become just as good as running back. Even crossing an ocean can happen on purpose or by accident in a storm on some uprooted tree.
Leaving the solar system on the other hand requires the cooperation and focused effort of thousands or even millions of people to finally send away some handful or dozens. It’s not an individual decision, not even the decision of some group or even a country. It requires to some degree the cooperation or at least consent of most of the worlds population. The costs are so enormous that everyone on Earth would feel the consequences, the drain of resources. For many poor or sick it might be the last straw, and for many rich really irritating unless they gain from it.
Contrary to conquer a foreign country or discover a new continent, there is absolutely nothing to gain from the trip itself. It takes centuries or millennia to get there, even more centuries or millennia to build up an economy on the new planet strong enough to organize a trip back, and again centuries or millennia to come home. And there is probably nothing out there that some socielty not has already in their own solar-system. Being able to build an interstellar space ship is proof in itself, that they have all they need. There are really only costs and no benefits (besides some entertainment, a little rather useless science and of course a chance for a few to get rich from diverting project-funds into their own pockets).
We can assume that the rules that govern the economy of an alien society are similar to those we have to obey to: Resources are always limited, valuable resources are wasted during development due to incomplete knowledge (in an initially uninformed trial and error approach), and their economy, as a whole, follows the usual patterns of evolution. Societies do in general not accumulate much surplus because either the society looses motivation to work until surplus is consumed, or surplus is stupidly wasted, or deliberately sabotaged, or stolen, or some lazy group lives from that surplus while the rest work for it. To create the resources interstellar flight requires, the society in question must either work harder or by other means produce more or consume less. And most of all, they need to be in agreement. Regardless the species, evolved groups are never in agreement, simply because agreement means evolutionary stagnation. In order to be prepared for the next unforeseen disaster, you always need to keep some doors open for different ways of thinking, living, having sex, earning money, doing research or spending resources. And if costs are high and expected returns equal zero, agreement ought to be hard to come by.
These arguments taken together are the reason that I do not believe, that there are no more than 1 in 1000 intelligent, technological societies that right now may atempt to organize an interstellar travel.
Anyways. We now assume that some alien society plans to send some of its biological individuals, robots or cyborgs to a planet outside their solar system. The destination planet may be a rock or a habitable planet. To calculate the costs they need to ask five questions:
A. How far do they intend to travel?
B. What technology do they have or not have?
C. Within how much time do they intend to arrive there?
D. How much stuff and/or people do they intend to take along?
E. How big are the chances for success?
A. Distance
If their goal is just the first and best dead piece of rock circling another sun a travel of a few light-years will be enough.
A planet with primitive life should be found within 10 light-years.
For a new planet to live on (with moon all), they need to travel at least 100 light-years. Chances are high that such a planet is already inhabited by intelligent or even advanced technological beings.
And if they want to visit, conquer or move in with their next high-tech neighbor that has interstellar aspirations, they need to make a trip of at least 1000 light-years.
B. Technology
When some aliens want to go somewhere in space, they need two things: a rocket and fuel.
The equipment they need to bring all the way to the new planet we call the payload, including some landing vessel, them and their belongings, the engine that decelerates them and so on.
Everything they use up and throw away during the journey we call fuel, including the fuel itself, used stages, empty tanks, unused spare parts, broken stuff etc. All that can be melted down and shot out improving thrust, thus turning into fuel. When they calculate speeds and travel times they look at 2 factors, the fuel-payload ratio and the specific impulse of their propulsion systme.
The fuel-payload ratio simply expresses how many kilograms or tonnes of fuel they have for every kilogram or tonne of payload.
The limiting factor of all space travel is the power of the engine that propels the rocket. To lift from a planet they need an engine that produces tremendous thrust for a short period of time. A rocket driven by chemical fuel is well suited for that. Once in space they need an engine with high specific impulse. What ever they shoot back, the faster it exits, the more it propels the rocket per unit fuel. And they need some fuel that releases a lot of energy for every unit of mass.
The ideal fuel would be antimatter. All matter is transformed into energy releasing horribly amounts of energy according to Einsteins equation E = mc². However, I rule out anti-matter engines for any alien society anywhere in the universe, simply, because one can not handle the stuff. Even if they manage to produce it, the slightest contact with the tiniest grain of dust results in an explosion, that tears their antimatter container apart, whatever it is made of. The chance to successfully transport big enough quantities into orbit and store them in some vessel, to use them during a flight that will last many years, centuries or millennia, without a single incident is virtually zero. And any society that is stupid enough to produce the enormous amounts of antimatter needed for a long interstellar trip is literary inviting the next best fanatic or clumsy intern to blow up the entire planet.
If they have an engine with 100% efficiency in its transformation of energy into thrust and a mass defect of 0,64%, that is the amount of mass of that is transformed into energy (for instance hydrogen that is fused to helium) their engine will have a specific impulse of 0,11 c (2) (c = speed of light). Some fusion or fission based engine with that specific impulse is theoretically possible. It could propel a rocket to a maximum of 45% of light speed if they intend to only fly by or crash into their destination at top speed, but only to 22,5% if they intend to slow down to zero upon arrival.
In order to calculate speeds and travel times I looked for equations and calculators. The space travel calculator found here tells us that flying 10 ly (light-years) with a fuel:payload ratio of 10:1 will take about 12 year measured back home, about 5 years measured on the ship. “Great! Lets go!” the aliens say, build a ship, fuel it, launch and die all on the way. Why? The calculator assumes constant acceleration. That is a rather bizarre idea. Imagine you had a large and strong, fully loaded, fully tanked truck climbing up a hill with a fuel-payload ratio of 10:1. Let’s say the engine accelerates that truck with 0,1g. Fuel is burnt at a tremendous rate and the truck gets lighter for every minute. Due to the falling weight acceleration goes up to 0,11g. But instead of shifting into second gear you rip out that strong engine and replace it with a weaker one, so you only have 0,09g. Again, fuel is burnt and after a while due to lower weight acceleration of 0,11 is possible. And again you throw out that engine and take a weaker one. This way you could have (kind of) constant acceleration. Alternatively you could keep that strong engine but throttle it more and more as the truck gets lighter. Why would our aliens do such thing? Well because the guys i mission-planing used those easy to come by constant acceleration equations and now they are stuck with them. Consequently the trip takes much longer and everybody starves.
However, an engine produces constant thrust, not constant acceleration. The ship of our aliens will be very heavy at start (just like a conventional rocket) and get lighter and lighter during the flight, until the end, where only the mere payload remains. That means that it will have a long and slow starting period and a rapid and short deceleration near the destination.
If you calculate with constant thrust, things look very different!
For all the following curves the blue curve represents a 1:1 fuel-payload ratio, the red a 10:1 ratio and the yellow one a 100:1 ratio. On the y-axis we have the speed of light where 0,5 would be 50% or 150 000 km / sec. And on the x-axis we have time as measured from the home-planet. The numbers from 1 to 10000 may represent what ever one likes, days, years, minutes or fractions thereof. In all cases, the engine produces constant thrust.
This diagram shows a non-decelerated speed curve for the acceleration phase of rockets with different fuel-payload ratios (3).
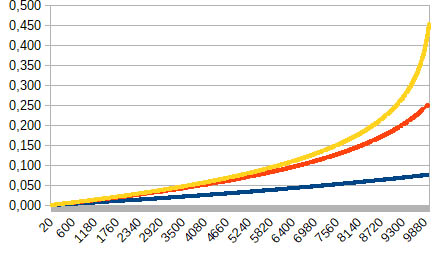
In order to have enough fuel to decelerate again, the ship needs to stop accelerating a long time before it reaches the non-decelerated top speed. The next diagram shows a decelerated speed curve for the acceleration and deceleration phase of rockets with different fuel-payload ratios. At the sharp peak one would turn off the engine and just cruise for some years or centuries.
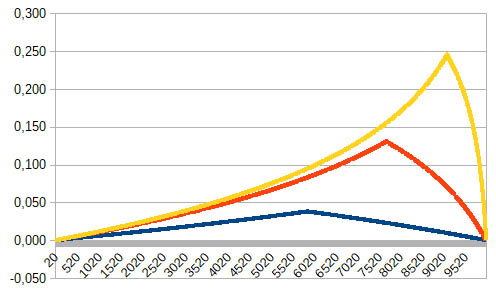
When talking about those higher fuel-payload ratios, one must bare in mind, that in order to burn 10 or 100 times the amount of fuel within the same time, you also need a 10 or 100 times more powerful engine.
A speed curve with cruising would look like this:
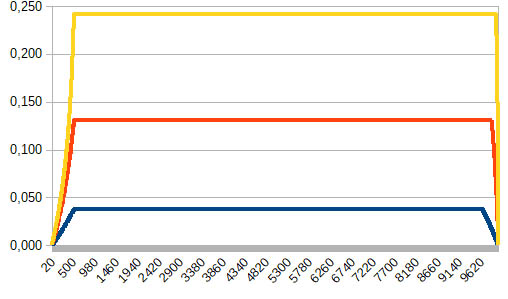
While there is a physical limit to the specific impulse, I assume there really are no limits we can take for given, when it comes to the actual power of the engine and how much thrust it can produce. A civilization with a sufficient amount of zest that wants to develop an engine should come up with something that is simultaneously light-weight and rocks.
Besides the engine there are probable limits to how big a ship you can build, mostly because material has to be brought into orbit. That requires resources of all kinds and resources are, as we know, always limited. Somehow I feel that a ship of 1 million tones is about the maximum of what a society on an earth-like planet could bear. At a 1:1 ratio 500 thousand tonnes would be fuel, the other half ship, tank, engine, stuff and passengers/crew. At a 100:1 ratio only 10 thousand tonnes would be payload of which most go to ship and engine.
C) travel time
If our aliens make a short trip of only a few light-years they probably want to go there as fast as possible. That means that they take their most powerful engine, step on it all the way and hit the breaks just in time.
If they make a long trip of some hundred light-years it really does not matter whether the journey takes 9 or 10 thousand years. So they burn most of their fuel the first few decades or centuries, then turn everything off, cruise for some millennia, and then decelerate when they approach their destination.
In any case, the speeds are so low, that there is practically no relativistic time dilation. That means that despite all the wonderful things we see in sf-movies and even learned in physics at school, they will age just as much as their twin brother back home, give or take some percentages or so.
That also means that their ship will age just as much.
The following table shows how far they can travel within a 1000 years of flight with different fuel-payload ratios, a powerful engine and a specific impulse of 0,11 c.
Fuel-Payload ratio one way | Distance traveled in light-years one way | Top speed in km/sec |
1:1 | 40 | 11 000 |
10:1 | 125 | 39 000 |
100:1 | 200 | 72 500 |
If they want to go home again they probably have to bring the fuel for the trip back, because there is no fuel where they are going or no way to get it to their ship. Top speed on the way out will then be much lower since the engine with constant thrust has to push that much heavier vessel. The speed-curve looks like this, with c on the y-axis and time on the x-axis.
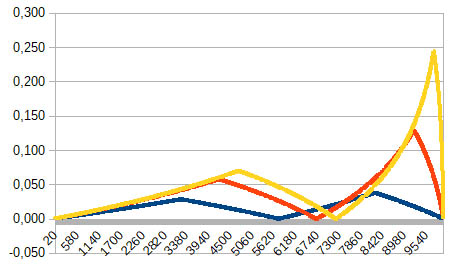
Fuel-Payload ratio at start | Top speed out as % of light-speed | Distance one way in light-years | Fuel-Payload ratio at start of return | Top speed back as % of light-speed | Ratio between flight time out and back |
2,35:1 | 2,84% | 8,1 | 1:1 | 3,8% | 1,35:1 |
30:1 | 5,78% | 19 | 10:1 | 13% | 2,06:1 |
360:1 | 7,01% | 24,5 | 100:1 | 24,6% | 2,6:1 |
Besides travel time, much time is needed for preparations. And those in turn depend on current knowledge, technology and goal. Do they already have explored their own solar system and want now to put a robot on the nearest dead rock before the end of the millennium, or have they just invented the wheel-barrow and want to move to a new home before the now unemployed god of back-pain takes revenge. Either way, they need some serious RnD and have to spend a certain percentage of they annual surplus on that project for the next many years to come.
D) The payload
There are generally two reasons why any society would attempt to send someone or something far, far away: Research and colonization, where the first may be a necessary step to succeed with the second.
If their goal is research they can send some light-weight measuring and communications equipment. Some kind of robot will do. A few spare parts. If the thing breaks or crashes on the way out or upon arrival it’s sad, but not a big deal. The ship they need can be small. Depending on the information they want to gather, they may not even slow down, but get there as fast as possible, take a few shots, measure some atmosphere composition or something, send back the data and get lost.
Fuel-payload ratio can be high because the mass of the payload is so low.
I’m unsure about communication equipment. To have any benefit from a mission one might want to know at least if that probe made it all the way plus those blurry images. For that our aliens will need powerful transmitters. I could not find any information on how big and strong a transmitter has to be to send a certain distance, besides a mentioning of a 100-light-year-boundary.
Colonization or even invasion is different. A lot of stuff is needed and one must make sure everything works upon arrival. If you look at those monster-ships in sf-movies there is usually virtually no room for fuel. There are rotating arms to ensure artificial gravity and the comfort of the passengers. There are troopers, weapons, equipment of all sort, bars, pools, chairs, tables, you name it. But no gigantic balloon-like tanks, several times the size of the ship. Reality would be very different.
For every extra kilogram from a pair of boots, a chair, a pet, some books, an ax, a bag of seeds, or even the paint on the wall they need between another 1 and 100 kilograms of fuel and accordingly bigger tanks. So they will think twice and three times if they really need that stuff.
“You want an entire room, with bed and shower for your privacy? Are your kidding, dear sentient being?”
E) Chances of success
There are basically four factors that can cause their mission to fail
a) design flaws, lack of knowledge, sabotage, disagreement, natural disasters, economic breakdowns etc before or during start or during flight.
b) wear and tear of crew, rocket or equipment during flight
c) collision during flight
d) unexpected problems upon arrival
These are the stuff good movies and books are made of. Since I do not intend to write a book here I will keep it short.
a) Anything can go wrong before they even get everything set up for departure, and this is already covered in section 2. Issues and motivation in highly technological societies. Here we assume that our aliens made it so far and that the ship is made as good as it can be, without any intentional flaw built in by some mad alien. However, intentional or not, even the best will miss the unforseeable.
b) Nothing lasts forever. Rubber dries out, metal cracks from fatigue, memory (in devices, DNA or where ever) deteriorates due to cosmic radiation, failures during backup or copying. Screws loosen due to temperature changes. People get on each others nerves, virus evolve over time, milk turns sour. While our aliens might have a good chance to make a 100 years flight (to the next dead rock), their chances to travel several thousand years (to the next suitable new home or even existing high-tech society) their chances are very slim. They may choose to shut down everything during cruise and hope they can start it up again millennia later, or they may keep some diagnostic, self-repairing process running. In the first case, chances are slim because during all that time something may loosen, crack, or break otherwise. In the second case they relay on some intelligent entity to keep its sanity. Such a diagnostic process needs a fair degree of freedom to respond to unexpected events, including failure of this process itself. And there is no way to make sure that this process will not deviate from its original design and orders. Just as any society develops over time, such a program will. Personally I believe that the chances of success from this factor alone are as low as a few percentages for any long trip.
If the risk that something vital breaks within one century is let’s say 5%, at a travel of 5% of light-speed (1:1 fuel-payload ratio) the following table shows the chance of success for longer trips. If you go faster, have a more powerful engine, bigger tanks etc, chances for success get rather lower than higher, because higher speed means more stress to the system (4).
Light-years of travel | Chance that nothing vital breaks |
1 | 98,5% |
10 | 85,6% |
50 | 46% |
100 | 21% |
200 | 4,5% |
300 | 1% |
500 | 0,05% |
1000 | 0,00002% |
c) Being hit by a grain of sand or drop of water when strolling at 3 km/h down the beech is different from being hit at 15 000 km/sec (5% of light-speed). A particle that measures about 1mm in diameter with a mass of only 1 milligram will impact their ship like a projectile that weighs 225 kg, shot from a canon at the speed of sound (1km/sec) (5). An iron ball of about 40 cm in diameter weighs 225 kg.
That grain of sand will turn into plasma of many million degrees upon impact, expanding in a cone and melt away everything in its way until it has dispersed sufficiently and cooled down. It has enough energy to vaporize 18 kg of iron (6). Vaporizing 18 kg of iron (or steel) allows that grain of sand to pass through 20 cm of steel as easily as a hot knife through warm butter. Holes in the hull are not healthy for space ships. Not to mention all the valuable parts of the ship that get damaged when this cloud of several thousand degrees hot iron vapor hits cables, pipes, tanks, processors, and sensors and melts, bends, burns, breaks or pulverizes them.
Our aliens might build a shield that might resist that, but grains of sand do not like to travel alone. And for each kilo of shield they need more fuel.
A single drop of frozen water weighs 0,05 g and has 50 times that impact, like an 11 tonnes canon ball at the speed of sound. That again would be like an massive iron ball 140 cm in diameter. Space ships do not respond well to that either.
Problem with these hits is that all their energy is concentrated on a tiny spot. And the speed combined with the extreme cold of space makes materials crack like glass even if they are very strong at room temperature.
If our aliens run into a cloud of hydrogen of only some thousand kilometers in diameter (tiny with interstellar measures) and with only some nanograms of hydrogen per cubic meter, it will have the same impact as the grain of sand multiplied with each square meter of the ships frontal area.
A dust cloud with only a few microscopic particles per cubic meter will grind their entire ship away in a fraction of a second.
What can they do about it? Well, nothing, really. They travel so
fast that they have no chance to detect those particles even with
the most powerful telescope or radar. And even if they could, they
can not move them out of their way fast enough. They could burn them
out of their path with a powerful laser, but that again would cost
not only fuel but the weight of the laser. And if only the tiniest
fraction of something hits the lenses of their laser, it will make a
mark, causing the laser light to bounce and burn away the laser
instead. Magnetic fields work only on charged particles and on short
distance. There is no way to change course at that speed and evade a
cloud or even a single particle.
I do not know how many grains
of sand there are in interstellar space, how much ice, how many dust
and hydrogen clouds. I guess nobody knows and nobody will ever know,
because any probe one could send will disintegrate upon impact and
one will never know why it suddenly became silent.
If they had a spacecraft with a 1:1 fuel-payload ratio and a frontal area of lets say 100 m², and the chance that they make it for one light-year without colliding with anything was 99%, chances drop dramatically, if they travel far.
light-years of travel | Chance of success with 100 m² frontal area |
1 | 99% |
10 | 90% |
50 | 60% |
100 | 37% |
200 | 13% |
300 | 5% |
500 | 0,6% |
1000 | 0,004% |
Even if the chance of success flying one light-year was 99,9% the chance to pass 1000 light-years of (not so) empty space still would be only 37%.
Now, if these chances where not already low enough, they even sink more if the fuel-payload ratio is high, because now the tanks need to be gigantic and the frontal area becomes much bigger, increasing the risk to hit something. If they want to go fast, using a ship with a 100:1 fuel-payload ratio their frontal area might increase 10-fold, decreasing those already low odds by a factor of 10. At 20% of light speed this tiny one milligram grain of sand suddenly can boil away not only 18 kg of iron but 290 kg. It easily gets through 55 cm of steel (or any other known material). Now a grain of only a 60 micro-gram would have that 225 kg iron boiling impact. 60 micro-gram is the weight of 1 cm of a human hair.
If they could survive the impact with such a particle at 5% light-speed, at 20% they can not. And if they enter a solar-system at that speed or even higher (as when they send a probe, not slowing it down upon arrival) chances are very high, they will never get any information back, because solar-systems are dirty places. Dust from comets that passed some millennium ago, dust from volcanoes, solar flares, bacteria, water droplets ripped from some atmosphere or eruption like we can see on Europa. At high speed, they hit one tiny particle and the party is over.
d) Unexpected problems upon arrival are highly probable. If they sent a probe some millennium ago, the data it sent back, is as many years old as that planet is light-years away. Lets say 1000 light-years. It will take them 5 000 years to get there with a 100:1 fuel-payload ratio or 25 000 years at 1:1.
Much might have changed during that time. Some primitive cave dwellers, that could have worshiped them back then, might have evolved to nuclear powers, not so happy to see them. Previously favorable climate and atmospheric composition can change due to volcanic activity, climate cycles or the newly evolved nuclear powers failing to keep their trigger happy fingers from trying to become the greatest and only power of the planet. And of course a thousand other unexpected, unforeseeable and potentially fatal things might go wrong. And usually do. However, I try to stay positive and simply guess the following odds, based on that our aliens can gather some information about a planet near by, but virtually non about one further away.
light-years of travel | Chance of surviving unexpected problems |
1 | 99% |
10 | 90% |
50 | 60% |
100 | 50% |
200 | 50% |
300 | 50% |
500 | 50% |
1000 | 50% |
So, what are the costs?
Traveling to an other continent costs here on earth about 2-10% of the annual income of a person with western standard of living. To put a man on the moon cost the USA about 4 % of their GDP over 10 years or $ 6,500 billion . One launch of a Saturn V cost $ 185 million (multiply that with 6 to get today’s money value). Going to mars will cost a little more than going to the moon. Traveling to a planet far away outside our solar system is an entirely different thing.
A Saturn V has a fuel-payload ratio of 60:1. Launch costs are interesting because fuel, rocket and cargo for an interstellar flight have to be brought into orbit and have to be assembled there. Assembly stations might be in place from earlier inter-solar-system expedition, but probably they are not, because research for interstellar travel took some centuries and the stations are outdated. Interplanetary missions will only continue as long as they are fruitful. Once most or all planets have been visited and investigated there will be no more reason to fly there. Resources are cheaper on the home planet. If there was, for instance, gold on some planet: At a ratio of 60:1 you need basicaly 60 Saturn V launches of 1 billion dollar each to bring all the material to that planet that you need to assemble one rocket to lift off again from that planet and bring something back. That does not include things that break and have to be sent twice, starting ramps, drilling- or mining equipmnet, personal, food, shelters, robots, computer, communication, medical supplies and spare parts etc. If you then can get back 1 tonne of gold that would be 60 million dollar per kilogram, compared to Earth gold price of about 50 thousand dollars. And that would be the price inside our own solar system. People talk a lot about colonizing mars, but I have a hard time imagining that we will put up more than a temporary tent up there. Moving to mars would be just as pointles (while far more expensive) as moving New York to the Sahara or all the greenhouses of The Netherlands to Antarctica.
And if our aliens plan to run with a 100:1 fuel-payload ratio and they intend to colonize or conquer some other world, they have to lift a tremendous amount of fuel into orbit. There are for sure cheaper ways, but there are always limits to how cheap you can make something while maintaining a high degree of safety, a low degree of vulnerability and a low environmental risk and impact. The energy needed to escape the gravity of a planet is constant.
If we take that 1 million tonne ship as some reasonable limit, all the stuff you need for colonization/invasion will only fit on a 1:1 fueled ship, and the trip to the next colonizable planet or invasionable society will then take eons.
I will not attempt to put a real price tag to all of this. There are, after all, to many vague assumptions and variables. But whatever costs one arrives at, they have to be multiplied by the number of flights you have to make to have at least a 95% chance of success (or something) . If there is, for instance, a 16,5% chance to successfully make one 50 light-year flight, you need to make at least 35 flights (log0,95(0,165) = 35) to have a 95% chance to succeed once. However, if the reason for failing to reach a certain destination is a permanent dust cloud the ships have to pass through, then no ship will ever make it and the chances of success are zero until our aliens find out where that cloud is, how big it is, how it travels in relation to their intended route and find a new route, free from any dust clouds. Depending on how far away that cloud is, that might take millennia to figure out. In this case one has to remember that it takes A LOOOT of fuel to change course during a filght at high speed, that sling-shots are impossible because moving at high speed through a solar system is probably fatal (see above). So zig-zaging around dust clouds and other obstacles will extend flight time and need of fuel by several magnitudes.
light-years of travel | Combining the odds (simply multiplying all the odds above with each other) | Number of flight to have at least a 95% chance of one single success |
1 | 96,54 % | 1 |
10 | 69,34 % | 7 |
50 | 16,56 % | 35 |
100 | 3,89 % | 63 |
200 | 0,29 % | 115 |
300 | 0,025% | 160 |
500 | 0,00015% | 260 |
1000 | 0,0000000004% | 500 |
So instead of a real price I will try to imagine how a society, alien or domestic, would tackle the matter.
After exploring their own solar-system the natural next step would be to send a couple of robots to the nearest solar-system where there might be some primitive life. With a sufficiently powerful telescope it should be possible to find a planet, that has an atmosphere where spectral analysis shows oxygen or something that only life can produce. If the distance is, let’s say 5 light years, the trip would take a few centuries depending on the fuel-payload ratio, this societies can build a rocket around. It should be possible to build a ship that keeps together for such a relatively short time. Risk for collision is low since the path can be checked with telescopes etc, and since the planet is close by it should be possible to have enough information to prepare for a large array of problems upon arrival, landing and the first weeks on the planets surface. Chances for success could be as high as 90% and costs for fuel, ship and equipment relatively low, may be not much higher than for a travel within the own solar-system. During travel, every decade or so, the population may be reminded of the enterprise, to keep interest alive. If the first mission fails one might even send a second, or third, learning from mistakes. Sooner or later the robot may land, make some discoveries about new life-forms, send back the data and everybody is happy. Since the planet is near by, some kind of contact can be kept, if the robots survive long enough. The chances for that are, however, not to high. If there is an atmosphere, there will be weather. If there is life, there will be corrosive chemicals produced by those life-forms and there is no way to predict what they will be like and how to protect the robots from them. To detect those chemicals and inform the home planet will be exiting stuff, but in their nature useless. The organisms producing them can not be sent back. Their kind of DNA might be understood, but usually are powerful chemicals easily produced without the need of exotic organisms, once their chemical composition is known. A mission to retrieve those chemicals or organism would probably be much more expensive that synthesizing them from scratch back home. Sf-movies give in this case a very wrong picture of the nature of physics, chemistry and most of all economy.
While the economical value of such a mission is miles lesser than zero, the entertaining, moral or philosophical-religious value may be high. Detecting that there is life, and that it equals or differs from life on the home-planet would spur plenty of research, discussions, may be even revolutions.
If might even spark a new expedition to another life-hosting candidate near by.
However, whether it might motivate the society to prepare the next natural step, to send some robots to the nearest planet where there might be higher life-forms, even intelligent life, is a different thing. The distance may be as much as 100 or 200 light-years. That alone would account for a travel time of several centuries or even millennia. The ship needs an entirely different protection to withstand decay over time. Risk for collision may be as high as 65%. There is virtually no information about the conditions the mission will meet, and most importantly, there is no way to actually identify that planet. The atmospheric composition of a planet of bacteria, algae and fungus is in no significant way different from one hosting fish, dinosaurs and apes. Especially if the nature of life on that planet is unknown. I doubt that it is possible to detect a large moon or a magnetic field over a distance of many so many light-years. It will be hidden until there is not only intelligent life but until some (careless) high-tech society starts to produce radio-waves, strong enough to be detected far away.
The only way to find a planet with higher life-forms would be to check all of the thousands of candidate planets one by one. That would not only cause costs several magnitudes higher than the cost for the first kind of mission, but also require a dedication to that mission that needs to last for many, many millennia.
It has been suggested to send very many very small probes, shot away from Earth at speeds close to the speed of light. However cheap per piece, the high speed practically guarantees that they collide with something they can not survive. At 90% of light-speed, the collision with something as small as a single bacteria is fatal for a small vessel. And even if there is only one single hydrogen atom in every cubic meter of space on a 100 light-year travel, the mass of all that hydrogen amounts to the mass of 1000 bacteria per square meter, wearing down the vessel whithin the first year.
And what could any society gain from such a mission. If we look at the millions of variations of life-forms we find here on Earth, it is highly doubtful that there are any life-forms out there that significantly differ from what we can find here. Unless, again, we talk of some high-tech society. That indeed, could be very exiting, a not because they may be very different from us, but because they might be similar in their thinking and feeling. And not because we would make friends, but we would become enemies (see Part Two). But to find something that can, well, fly on wings, swim in water, or run on ground is not worth the effort, even it it has ever so large and ever so wonderful pink eyes.
The extreme costs also rule out that find-a-new-home-because-our-planet-is-dying-from-our-polution-argument. It is and will forever be much cheaper to empty the trash can than to build a new house. So it will be cheaper to clean up the mess, than to attempt to find a new suitable planet, and move that (stupid, thoughtless and messy) population over there, just to kill the next planet and all its life-forms.
4. Pre-existing life on probable target planets
Since we target a planet where the atmosphere is already breathable, let us consider what kind of life we may find and meet on that new world. Even for simple life like bacteria, the internal systems and bio-chemical mechanisms are insanely complex. For evolution to occur the information to construct these mechanisms must be stored somewhere and passed on in a slightly changed manner to the next generation. There might be any number of ways to do that, but since amino acids, sugars, and other organic compounds are found in meteors here on Earth, we can assume that they are present on other planets as well. And since strings of amino acids work so overwhelmingly well here, they probably do work somewhere else as well. Some sort of RNA or DNA kind of memory should be the predominant form throughout the galaxy. This should be good news for everyone who wants to colonize new planets, because food can be found. However, this is just as good news for every bacteria and virus on the new planet that wants to feast on alien flesh.
Although alien life might be similar, it will hardly be identical. The chemical profile on each world has probably shaped and determined what amino acids are used to code information. Alien life might just as well use different pairs, and more or less in number. Protein from two different worlds is most probable very different from each other. It might contain poisonous amino acids and metals like arsenic that make them useless for a settler. The bad news for a settler is that while primitive life easily adapts to strange new things, complex life does not. The body of a colonist might get eaten alive, while the immune system not even realizes that it is attacked, simply because it does not recognize the virus or bacteria as something dangerous. Bacteria- or fungus-like things may break down the alien protein as it would any other pile of something half-rotten organic. And since there are millions of different species of bacteria and virus that will try to get their share of the feast, but only a handful of colonist are around to fight them, probability is high that they do not survive that long.
Then of course instead of sending biological beings one might send robots. But sending robots to a planet full of life will work just as bad. Dirt, bacteria, rain, mud, lightning, dust, corrosive fumes and what-not are just as destructive for robots. How long would our best robots survive without maintenance in the African jungle for instance?
A robot can of course colonize a lifeless planet. But how much fun is it to spend half their economy to send a dead thing to a dead rock in hope that this robot might turn that dead rock into a not so dead rock during the following thousands of years of terra-forming.
And anyhow, when they design the robot they have to compromise between durability and repairability, power and power consumption, flexibility of mind and rigidness to follow a program. Whatever choice they make, the robot will have some weakness for each strength. And if it is alone several light-years from the nearest hardware shop it can not afford weakness. A long time before this robot has succeeded to produce its first new solar panel or battery or only the raw material to make them from, it will have stumbled over a rock in some sand storm and broken an unreplaceable manipulator or simply fallen into some pit.
5. Consider the consequences!
When considering to colonize a new world, our aliens must send people or robots that are ready to kill, fight, struggle and to do what ever it takes to survive against all odds. They send their worst. They send the guys that stop at nothing. Those that think fast and shoot faster. Those that don’t care if they step on a precious first-flower-ever-on-the-new-planet or the only-other-intelligent-species if they feel the slightest threat. They send them there with the ability to adapt, to multiply, to conquer, and to make this new world their world.
They can either send them to a dead rock and let them terra-form for millennia or to some place where most of the work is already done by bacteria-, plant- and/or animal-like things.
In both cases they need to realize, that the colonizers will change during their time there. Adaptation means evolution. Evolution means divergence from the original objective. In sf-movies robots carry out the same stupid tasks for years and years. In reality, if they build an intelligent robot, able to adapt to circumstances they know nothing about when they send it off, this robot will need to be able to rebuild and reprogram itself thus replacing any original order or instruction that might turn out to be counterproductive. If they choose to send them to the dead rock, they do not need to be killers, they can even be real nice, but they need to be there for a very long time. And the longer they are gone the stranger they might become.
When the European countries started to colonize other continents, the kings and queens back home could keep control for a few decades or cenuries before the colonies declared independence. Should we humans send troupers to some new world, there would be no control from second one. Once they are gone, they are the new kings. Combine that with their dubious character and a long time of evolution and they do not colonize a new world, like “we humans just opened our new branch on Gliese 581 d”. No! We create humanoid aliens! Compared to the folks back home, the colonizers will evolve rapidly and in unforeseeable directions. And not only are they not, well, just a bunch of nice aliens from that planet down the road, no, they are the really tough and aggressive sort. They know all about us, know our weak spots, “have detailed files”. And if they for some reason start to dislike their new home, the old home will be their first target. They can, of course, come back in peace, but then again, they might not. They might send some well designed virus first, to get rid of the part of the old population they never really liked anyways. So before we send someone or something out there, we ought to consider twice.
A colonization of more and more planets would only be meaningful, if there was such thing as a “stargate”, “far-caster”, “teleportation portal” or whatever one could call it. Home could stay in touch, travel costs would drop to virtually nothing, one could send a robot to the nearest dead rock, set up that thing, or let it float in space, send a new ship through the portal, carrying a new rocket, with a new portal, and even send information and valuable material back home. But since such a device would demand a fair amount of physical lawbreaking, I don’t think it can exist. And, the curious fact, that we apear to be all alone in this galaxy supports the idea, that no aliens have discovered star-gate-technology either.
Another scenario in which I could envision a “society” to send a mission far, far into space, could be “the aging AI”. On some planet there might evolve an AI. After millennia of gaining knowledge and power, after exploring physics and all other forms of science to their limits, having conducted all possible experiments with “lower life-forms” it might just get sick and tired of its home solar-system. It makes some very accurate calculations, sending out probes to pick a fairly safe path through the nearest some thousand light-years, builds a rocket that is sufficiently self-repairing and slow enough to survive the most probable kinds of impact, packs its stuff, puts itself to sleep and zigzags through space, from one solar-system to the next, waking up, every 100 000 thousand years or so, if something interesting shows up. It would not intend to ever come back. It would not even care, if it crashes into some unexpected comet or misses some slingshot and hits the sun instead. It had its thousands or millions years of exiting but lonely life, and just wants to see something new before it goes to heaven or hell or what ever it might hope for.
Such an AI might even visit us. However, the chances for that to happen are probably virtually zero. Not because it might not pass by, but because we are probably not worth stopping for.
However, turning back to our initial idea of some unstable, divers and therefore unpredictable alien society, loaded with all kinds of nut-heads, even if they are aware of all the calculations above, we can still imagine that someone would come with the following suggestion:
“I have this great idea to drain our society from all resources and force everybody into centuries of poverty and hard labor, in order to send a couple of guys away on a long journey. They will not have arrived by the time we are old or dead, but maybe our grand-grand-grand-children will eventually receive a message that they have arrived, if they survive the trip, which is highly doubtful, because a fusion reactor seldom can run for even a few years without incident or massive repair, and we are not sure if the crew and the computers can withstand the centuries of cosmic radiation, and there is no room for spare parts, and there is a 99% risk that they hit a gas cloud or some grains of sand in mid flight. And the crew will be old when they arrive, probably too old or too damaged to reproduce. But, hey, with some luck they don’t crash into a grain of sand and their radio still works when they arrive and every 100 years or so we can get an answer to some question we sent them. Would that not be something!!”
“Anything we can earn on that”, someone may ask.
“Well, of course. If we repack and sell the idea, running the program, we can skim our folks down to their blood and get rich here. But the planet we choose is so far away, that it can take some thousand years or so before they even can send back some boring rock.”
“Great! I like that skimming our folks part. If we replace all the high quality parts with some cheap crap we can get on e-bay, we can get even richer. Let’s dig into the project!”
Part 2 – Say hello … and goodbye!
What if, by chance, a high-tech society, capable of interstellar flight, lived near by, let’s say within a 50 light-years. How should we tackle that matter?
The chances that it is a peaceful society is, as we concluded above,
zero. So, they are not peaceful. They are somehow like us.
Troublesome, unreliable, changing. If we send them our coordinates
and some basic biological facts, what can they do with it?
First,
they will probably not understand.
The idea that mathematics could serve as a universal language might just as well be very wrong. The math of some aliens might foremost try to tackle change, complexity and chaos where the simplest expression is “a turning into b”, and where a and b are movements, not values. The entire idea of a single constant value might be strange to them and the expression of a = a be viewed as rigid and dangerous.
Or their mind might be focused on dominance, only interested on which of two values is bigger/stronger, and where no single value is definable without comparison to another and where the idea of two equal values is a horrible insult and imidiate declaration of war.
Or their math may view the world as ever merging and dividing groups and some a = a does not make any sense at all.
I personally believe that our difficulties to formulate a unified quantum-gravity theory comes from our faulty math. We are trying to build an oven from snow, so to say. A technological advanced alien society may be successful just because they chose a different math-path from the beginning, making our math entirely incomprehensible for some aliens.
And even if they do have a similar math and understanding of the world. Any way to establish a fruitful communication is inhibited by time and distance. If they send us anything, why should they be truthful. Neither we nor they have any reason to supply each other with any useful information until we can confirm that their intentions are peaceful. But we will never be able to to that. Even if we got a message, telling us that they want us no harm, and even if we believed that, a century will have gone by before they get our answer. Who knows in what state of mind they are then. So much has changed on Earth during the last 100 years. Wars we have fought, philosophies have come and gone, strange leaders we had and have. The lines between sound thought, vague belief and plain lunaticism are very thin. The mere discovery of a neighbor might spur any paranoid mental, religious or political development. They might misinterpret our message in any way. They may come to the conclusion that we are hostile. And for good reasons. The cost to destroy your neighbor in space is only a tiny fraction of the costs to defend yourself. And if you live in the constant fear of an imminent attack you can not defend yourself against, a preemptive strike is the best thing to do. Since both parties think that, and there are no ways to establish diplomatic relations, it’s best, you strike first.
If they want to destroy our civilization, no fancy exotic bombs are needed. If they send away a 2,5 ton heavy block of iron (like a heavy car), not decelerated, at a fuel-payload ratio of 10:1, it will reach 25% percent of light-speed. The energy released by such an object when it hits Earth is equivalent to the destructive power of the total global nuclear arsenal,
Keeping it in one piece or a few smaller ones, would cause major destruction where ever they hit. At that speed it would pass through our atmosphere within a millisecond, or maybe it would explode some kilometers above ground, releasing all its energy in a heat- and shock-wave that would destroy much of our fragile infrastructure over an enire hemisphere. Or it could hit a grain of sand some million kilometers out there and instead engulv Earth in a fireball. In what ever form it hits, i would distroy plenty and affect our climate for decades to come.
To get one of those objects into orbit and away to us, they only need a single Saturn V-like rocket. It can bring about 100 tonnes into orbit, enough for the object and fuel for a 10:1 ratio or even higher. They don’t even need any block of iron, the rocket or rather its engine and tanks alone would serve as destructive projectile just as well. On the other side, that block of iron would make some good protection against small particles of interstellar dust. If they attack us from not to far away they can launch enough objects to have a good chance that at least a few make it all they way, for relatively low costs. If “they” are humans or robots we sent away some time ago to colonize somewhere nearby, they would even have rather detailed information where and when to hit us for maximum effect.
An object that small is not easily detected, but if we do, let’s say, when it is as far from us as the sun is, we will have 40 minutes to launch some counter measure. For instance a missile. If we want to intercept an incoming object at some safe distance, we need to put up a spherical defense parameter all around Earth. While this object had years to accelerate we have only those 40 minutes. A Saturn V could bring maybe 10 ballistic missiles and some communication and aiming devices into orbit. Those missiles can reach speeds of 23 km/sec. To intercept the object in space, the distance between satellites from which our counter-measure may be launched must be small enough to meet the object at least at that parameter. At 23 km/sec our missile will travel about 50 000 km in 40 minutes. So the mean distance between satellites must be no bigger than twice that, or 100 000 km.
If they send the object around some sun in a slingshot maneuver or if they have allready built some outpost on another planet we will have no way of knowing from which direction it might come. If you want to defend a border, you can basically place one soldier for each attacking soldier along that line. But if we want to put up some defense parameter around our planet, we have to take into account that the area of that parameter increases in a quadratic fashion if we increase the radius.
For a parameter of 1 million km (three times the distance to the moon) we would need 1250 satellites.
An object traveling at 25% c passes 1 million km in about 15 seconds. Its momentum is so incredibly high that even if we hit the object, the debris/heat cloud will still hit Earth.
In order to improve our defense window to 2 minutes instead we have to extend that parameter to 10 million km and 125000 satellites. Maybe then we could push it out of collision course with Earth, or it could disintegrate and disperse suficiently upon impact with our missile. If we are able to hit it. If our missile needs to be within 20 m in order to destroy or sufficiently push the object, not only do we need to hit that tiny spot from a distance of some thousand kilometers, we also need to trigger the bomb within the tenth of a microsecond the object needs to pass by.
But if that object puffs out a 100 kg of sand, some time before it reaches us, so it is surrounded by a cloud of, let’s say, 2 km m in diameter, there will be about 25 grains of sand per cubic meter to grind away all missiles, we might manage to send. We already know what a grain of sand does to an object it hits at that speed.
And if the projectile splits up, we have not even a clear target to point our missiles at.
To sum it up:
We have no chance in hell to defend ourselves!
Conparing the costs: it takes 1 Saturn V launch to put 10 fully fueled and armed missiles into orbit, so it will take 12500 launches to build that totally ineffective defense shield.
It will take only a 100 launches to try to hit us first in a
preemptive strike. Each launch carries 4 bombs, giving us 400
chances to hit that alien planet. If the frontal area is only 1 m²
chances most of them make it all the way are pretty good.
At
25% c i takes about 400 years of travel to reach the target planet.
The engine only needs to work while accelarating if initial
calculations to hit the target are sufficiently accurate. The sack
of sand can be pushed out just when the engines are turned off. This
way the sand works as a shield all the way. Only very primitive and
durable course correction motors need to be working all the 400
years untill arrival. Very little can go wrong.
Any rational alien society with a DOD will come to the same conclusion. Sooner or later they would have a government (or some freaked out rich guy) that wil lissen to their DOD, and if it is only to get the contract to build all those rockets.
Conclusion
The upshot of all this is that it takes enormous resources and centuries or millennia of preparation and travel-time to a distant, inhabited solar-system.
Trying to colonize new worlds is a really bad idea: Worthless, expensive and probably unsuccessful. And if successful, dangerous.
If we detect an alien civilisation and attempt to make contact, we sign our doomsday-papers. And theirs.
My conclusion is that, sadly, successful deep interstellar exploration and colonization will forever be the stuff of great science fiction, but never of reality,
Lucky for us, the chances that an aggressive society nearby manages to settle their internal fights, puts their wit together and succeeds all the way through the process of taking to the stars just around now is extremely low. Its almost a paradox. The more aggressive they are, and the more powerful their rockets and weapons, the bigger the chance they blow up themselves instead.
So the good news is, we will never meet a Xenomorph or Harvester. And if they come anyhow, there will be maybe a handful. They will be in really bad shape after ten thousand years on ice and exposed to hard radiation. They will have the same trouble as we have entering the atmosphere. They will not have magically hovering spacecrafts and therefore no chance to get off again. They will have no guns heavier than the ones the nearest street gang carries, so they will get mugged by said street gang before they can blink… if they blink. Left in the gutter, they will catch a cold. They will then be found and treated with a hot soup by some nice old lady that finally and surely will kill them, not being adapted to our phosphorus based protein. And if they manage to call home, before they pass out, it will take 10 000 years before backup arrives.
While it is useful to search the skies for alien life, it is rather counterproductive to send probes, or even messages, telling some wiser and quieter neighbor, where we are and what we are.
But still. Even after having checked and double checked each argument, each equation, each number, I can’t let go of that vision of a future, where we and “they” meet on some distant planet, exchanging strange stuff and thoughts and culture and diseases. I can not imagine our wonderful universe being a place of a million lonely islands.
Appendix – calculations and equations
(1) The number of planets within a 20 light-year radius is about 150.
The volume of a sphere is calculated using V = 4/3 pi r³ (where pi = 3,141…, and r is the radius, in this case 20 light-years).
V gives you the number of cubic light-years (ly³) in this case about 33500 ly³. There are 150 suns per 33500 ly³ or 0,0045 per 1 ly³. If you calculate a new Volume V from a different radius (for instance 1000 ly) and multiply that result (about 4 billion) with 0,0045 you get the number of stars within that radius (about 19 million).
(2) Calculation of specific impulse from mass defect and engine efficiency
Thanks to DrStupid from the PhysicsForum:
“Let’s assume a small amount dm of fuel is “burned” with the mass defect d and the released energy is used to accelerate the exhaust with the efficiency η. If dm is sufficiently small compared to the total mass of the rocket, this results in the total energy
dE²=[(1−d)⋅dm⋅c²+η⋅d⋅dm⋅c²]²=(1−d)²⋅dm²⋅c4+dp²⋅c²
of the exhaust and therefore in the specific impulse
dp/dm=c⋅√[2+(η−2)⋅d]⋅η⋅d
With a mass defect of 0.64 % for the fusion of deuterium to helium and an efficiency of 100 % this results in a specific impulse of 11 % c.”
All speed curves have been calculated using the equation I got from DrStupid and others of the PhysicsForum. I’m very grateful for their support and time they gave me to answer my questions and help me understand the physics of all this.
dw=(1−w2/c2)⋅um * dm * √1-w²/c²
Where dw is a change in velocity as measured from an inert observer (not the ship itself), w is the current velocity, c is the speed of light and dm is the change in mass, due to the fuel that is burned. The entire term √1-w²/c² is the so called Lorentz factor that adjusts values like mass and speed to relativistic values. (You can see that this term goes to zero as w approaches c, so that even dw goes to zero, regardless how much fuel is burned, preventing the spacecraft to ever reach or even exceed light-speed).
I set up a spread sheet with 10000 steps in time as measured by the observer, burning a constant amount of fuel dm in each step, calculating the resulting change in speed dw, and adding or subtracting that from the current speed w. Therefor all curves show values from 1 to 10000 on the x-axis. Using c = 1, calculations become much simpler. The points from which to start deceleration in order to arrive a the destination with a speed of zero are located in time when the spacecraft has reached half of the top rapidity (speed measure on the ship, that can exceed light speed due to relativistic time dilation) it would have had at the destination if it had not decelerated.
Using the equation above you can calculate speeds for all kind of fantastic spacecrafts as I thought I would need to. However, my research and calculations for this article showed that the realistic (not to be confused with relativistic:-) speeds are so low that non-relativistic calculations would have been almost as accurate, give or take some percentages, that anyways do not matter, since half my values are educated guesses at best. Like 0,11 for the specific impulse of the engine. Using a more realistic value for the efficiency, let’s say 80% instead of 100%, would have decreased all top speeds by 20% and increased all travel times by 25%.
(4) Calculating probabilities
If you have a dice with 6 sides, chances that you get a certain number is one out of six or 1 divided by 6 (1/6). If you throw the dice twice, chances that you get a certain number twice is 1//6 * 1/6, because there are a total of 6 * 6 = 36 combinations and you want one of those 36 or 1/36 or 1/(6*6). You can also write that as (1/6)². If you throw that dice 100 times and you want to know the probability to get the same number each time you take (1/6)100.
At a speed of 7,6% of c one would travel 3,3 ly in 100 years. If the odds are 95% that all goes well on that trip than the odds are x3,3 = 0,95 or x = 0,95√3,3 = 0,985 or 98,5 % that all goes well on a 1 ly-trip.
(5) Calculating energy from speed and mass
The equation for that is: Energy (in Joules) = ½m*v² where m is mass in kg and v is velocity in meter / second. Now we want to know how much energy there is in a grain of sand that has a mass of 1 milligram, traveling at 5% c.
1 milligram = 1/1 000 000 kg. The energy in that particle equals
0,5 * 1/1 000 000 * (0,05*300 000 000)² = 112500000 J.
How much energy is that compared to an object traveling at the speed of sound (like shot from a gun)?
0,5 * m1 * v1² = 0,5 * m2 * v2² // we divide with 0,5 on both sides.
m1 * v1² = m2 * v2² // we move v2² over to the other side
m1 * v1² / v2² = m2 // we put in the values
1/1 000 000 * (0,05*300 000 000)² / 1000² = 225 kg or 225000 gram
(6) Vaporizing iron
We have 112500 kJ in our little grain of sand, able to vaporize 112500/347 mol of iron = 324 mol
mol to gram: 324 * 56 = 18 kg.
It takes 347 kJ to vaporize one mol of iron. One mol of iron equals about 56 gram.